Fossils of Our Genetic Past Like
paleontologists working in miniature, scientists studying the human
genome sift through our DNA to find relics of past viral invasions.
John C. Cannon goes along for the dig. Illustrated
by Emily Harrington and
Kristy Whitehouse. Illustration:
Emily Harrington Scientists
who study how our genes evolved have no fossil record to explore.
Helical bits of DNA dont leave discernible impressions in rock, as
do the bones of dinosaurs. Instead, clues about how our genome has
changed over time and how it functions today lie within the code
itself. Some of the most telling recent evidence comes from the
remnants of ancient invaders, called endogenous retroviruses. These
bizarre viral vestiges now reside deep within our own genomemillions
of years after they attacked the cells of our distant ancestors.
Termed endogenous because they are fully
incorporated into DNA, these retroviruses remind scientists of
fossils because the first ones penetrated our genome some 60 million
years ago. Their descendants now make up a staggering 8 percent
or more of our DNA. But the fossil analogy doesnt do justice to
these retroviral foes since their invasions. Like the ancient coelacantha primordial
fish that has existed for hundreds of millions of years and is
similarly called a living fossilendogenous retroviruses have continued
to evolve, sculpted by selective forces over the millennia. But
theyve remained remarkably similar in form to their ancestors, just
as the coelacanth has, and have changed little since entering our
DNA. It's a strange partnership. Imagine
if the ancestors of animals alive today had plucked the bones of
dead dinosaurs from the ground, inserted the bones into their own
bodies and, with slight modifications, used them for some new
internal function. Moreover, the animals then passed this new bone
and the function to their young. In effect, thats one way endogenous
retroviruses have interacted with our genome. Its
a window on evolution in action, says David Haussler, a genomicist at UC Santa Cruz.
This is emblematic of the type of innovation that must have occurred
in wave after wave over the evolution of vertebrate species.
Haussler and his colleagues at UCSC, including
postdoctoral researcher Ting Wang, have discovered that endogenous
retroviruses may have been critical to our development as a species.
Itinerant by nature, retroviruses apparently helped copy and rapidly
spread critical sequences that regulate when dozens of other genes
turn on or off in our cells. Such a mechanism could explain the
relative speed with which organisms diverged from each other to
form new species. Its a symbiotic relationship because endogenous
retroviruses offer the host a way to accelerate evolution, says
Wang. Theyre evolutionary reservoirs. Harboring the enemy A lot of our DNA is derived from infection, says
virologist Robin Weiss of University College
London. Charles Darwin would have been amused to learn that were
descended from viruses as well as apes. Weiss
was one of the first scientists to champion the existence of
endogenous retroviruses. In 2006, he wrote a paper commissioned by the journal
Retrovirology that reviewed major events in their discovery.
The scientific community wasnt convinced until advanced molecular
biology techniques like gene cloning and microarrays provided hard
evidence for endogenous retroviruses, Weiss observes, though
biologists first hypothesized their presence in the 1960s. Vertebrates have been faced with these retroviruses
ever since we came out of the waters, Weiss says. Their fate and
their continuing influence today represent an ongoing interaction
between genetic elements that invade us and how we handle them, he
adds. Like marauding aliens from science
fiction, endogenous retroviruses initially infiltrated our genome
by infecting reproductive cells. If the attack didnt kill the egg
or sperm, the host passed the intruders genetic material from one
generation to the next, unnoticed. To the viruss benefit, this
absorption into the hosts genome ensured its DNA would survive under
the management of the hosts cells. The
most notorious of todays retroviruses is human immunodeficiency
virus (HIV), which causes AIDS. A retrovirus like HIV is exquisitely
programmed for one mission: to slip past an organisms cellular
defenses and use the machinery of its adversary to make identical
copies of itself. Biologists think the precursors of endogenous
retroviruses also were virulent pathogens when they first entered
the genome. Unlike other viruses, however,
a retrovirus doesnt carry its own double-stranded DNA. In nearly
all living things, DNA is the template used to make RNA, the messenger
that tells a cell which proteins to make and how to stitch them
together. This mosaic of proteins creates our individual
traitsdifferences in eye and hair color, height and body type, and
other genetically controlled physical characteristics. But a
retrovirus packages genetic information in thrifty, single-stranded
RNA and relies on a special molecule capable of reversing the process
to make DNA. Hence, the prefix retro. When
the burden of the copying invaders begins to take its toll on the
host cell, a retrovirus has no qualms abandoning the cell for dead
to find a new genetic proxy. And it leaves behind a path of
destruction that can give us a cold, a flu, orin the case of HIVa
ravaged, nonfunctioning immune system. Most
retroviruses attack ordinary body cells, like the ones that form
lung tissue or the living layers of skin. Such assaults endanger
the host and are likely to harm the body. But the host wouldnt
pass the infection onto their offspring unless the retroviruses
managed to break into germ cellsthe sperm cells and eggs involved
in reproduction. The first retroviruses
that eventually became endogenous happened upon some of those germ
cells, bursting with their own genetic heritage for the next
generation. After such an infection, the cells duplicated the
foreign DNA. As they divided to form new organisms, virtually
identical copies ended up in every cell in the newly developing
body, including the germ cells. And so the cycle continued.
The strategy was marvelous, if completely
accidental, as in all evolutionary changeeven though one might think
the retroviruses had that intent all along. Remember, its the blind
watchmaker here, Haussler says. The endogenous retroviruses had
no clue as to where [they] should go. Regardless, the retroviruses
ensured the survival of their genetic information. But an overzealous virus can overwhelm its host,
killing it and leaving the virus once again with the predicament
of finding another genetic copy machine. It makes sense that viruses
would evolve to allow their host to survive while still churning
out hereditary facsimiles. Absorption into the hosts genome was
one way to negotiate that treaty. Once
protected inside the hosts cells, the retroviruses no longer needed
to waste resources building new protective coats of protein around
themselves, which all other viruses need. Nor would they have to
search for other hapless hosts when the original cells wore outa
dangerous undertaking that invites counterattack from an organisms
immune system. Saddled with these imposters,
the hosts adapted in a bizarre and seemingly risky way. If they
couldnt rid themselves of the deadly bugs, at least the organisms
could relegate them to a harmless statusor so the evolutionary
strategy worked out. This development benefited the retroviruses
as well, as the hosts stayed alive and healthy. At some point, however, the hosts stopped merely
controlling the retroviral DNA. New evidence shows that host cells
co-opted these relics and used their nimble mobility to increase
the power of certain molecules that dictate the actions of hundreds
of our genes. The whole idea that genomes
of infectious agents can be adopted by the host theyve invaded and
put to use is a curiosity, says Weiss. Many endogenous retroviruses
are highly mobile pieces of DNA called transposons,
which Nobel laureate Barbara McClintock discovered
more than 50 years ago. She contended that transposons have
far-reaching effects on how genes behave, a fact only later confirmed
by advances in molecular biology at the end of the 20th century.
By turning the tables, cells took advantage
of the jumping bean nature of endogenous retroviruses. Consider a
graphic designer, who might use a template for a webpage to create
the same basic design from scratch over and over. In the end, she
produces a variety of websites that resemble each other only slightly.
In the same way, cells may have pounced on the opportunity to copy
a desirable sequence contained in a stretch of retroviral DNA and
then distribute it throughout the genome, resulting in myriad uses.
In some areas, that splice fell into obscurity. But in others,
particularly near active genes, the sequence developed a role in
gene behavior. Many of these transposons
dont code for genetic traits, but they are excellent places for
certain molecules to attach and manipulate the functions of other
genes. These proteins control how genetic instructions contained
within DNA are transcribed onto RNA molecules. One of the key players among these proteins is
p53, a master regulator of the human genome. According to Ting
Wang, p53 is the most important molecule for humans. It's akin to
a cellular mechanic charged with repairing damaged DNAso important
that if it malfunctions, cancer commonly results. But it took
detective work by Wang to recognize that leaping retroviruses had
anything to do with this crucial genetic kingpin. The interlopers influence
Wang doesnt study viruses by trade. He doesnt work with highly
infectious diseases on a daily basis, and there are no white
contamination suits in his closet. But four years of bench research
after college gave him intimate knowledge of wet genomic research.
In graduate school, Wang traded his pipettes for computers, completing
his doctorate in bioinformatics. Now a Helen Hay Whitney
Fellow, he spends much of his time in a tiny partitioned cubicle,
surrounded by computer screens. There, as a crack bioinformaticist,
he develops new algorithms to comb the human genome for new
information. Although Hausslers researchers are known internationally
as intrepid explorers of the genome, the work with p53 is their
first foray into the world of retroviral invaders. To enter this arena, the team had to go beyond
microarray technology, the standard genomic research tool of today.
In these experiments, tiny magnetic particles carry highly specific
probes to seek out and signal the presence of specific genes in
long strands of DNA. Its a technique Wang often used during his
bench research. But because microarrays
explore only certain areas of the genome, this search method is
limited. Genes that carry instructions for proteins only make up
a small fraction of human DNA. Most of the rest90% or morehas been
denigrated as junk DNA. Before searching for a gene of interest,
biologists use a chemical treatment to mask stretches of repeated
sequences of DNA, which are thought to contain few genes or other
segments of interest. If those segments arent masked, the microarrays
probes could be swamped by hits that biologists have often presumed
to be meaningless. What is all this
junk? Its a hotly debated subject in genetics today. Keith Garrison,
postdoctoral researcher in immunology at UC San Francisco, says
scientists are just beginning to comprehend what lies in the stuff
between our genes. The assumption has been that its junk DNA, but
I think thats an assumption you make at the peril of misunderstanding
what a large proportion of the genome is doing at any given time,
Garrison says. He and his colleagues are trying to develop an HIV
treatment by tapping into part of that vast, unknown reservoir (see sidebar). Theres
always treasure in junk, Wang agrees. These sequences are there
for a reason. The treasure
within The connection between
certain portions of junk DNA and the master regulators of our genome
began to crystallize for Wang in 2006. A rival
team from Singapore detailed the locations on the genome where
p53 can bind to strands of DNA and exert its controlchemical landing
pads that scientists call binding sites. But in their efforts to
determine exactly how p53 behaves, the Singaporean scientists ignored
the sites buried deep within the repetitive junk sequences. Wang read the paper in the esteemed journal
Cell. Something just hit me, he says. I thought, What
if this is something biologically meaningful? Applying the data from the Cell paper,
Wang examined the occurrence of p53 sites using the Haussler labs
powerful genomic browserscomputer programs designed to find specific
bits of DNA among billions of possible matches. He then compared
what he found to the sequences of known endogenous retroviruses.
To his astonishment, one-third of p53s binding areas sat squarely
within patterns of retroviral DNA. That many binding sites associated
with such an important molecule suggests these fossils arent just
remnants of past infections. Rather, they are more important players
in the genome than anyone imagined. The team published its findings in the Proceedings of the National Academy
of Sciences. We have many genes in the
genome that are master regulators like p53, where one gene controls
a lot of other genes, Haussler says. So how did they establish
their empires? The answer, he believes, is the curious penchant
of endogenous retroviruses to travel and spread within the genome.
The alternativethat these binding sites for gene regulators arose
by chance in many different places in the genomestrikes Haussler
as implausible. That would take an enormous amount of evolutionary
time, he says, too much to explain the colorful diaspora of living
things we see today. That puzzle catalyzed
Hausslers lab. We just launched a whole bunch of computational
work to pinpoint: What kind of endogenous retroviruses are they?
How old are they? And when did they enter the ancestral genome?
Wang says. All mammals have p53, from mice
to humans, but, mysteriously, the molecule can act differently from
one individual species to the next. The evidence from Wang and his
team points to the entry of endogenous retroviruses as one reason
for this variation. Two families of endogenous retroviruses where
p53 is particularly active entrenched themselves in our ancestors
genetic makeup some 25-40 million years ago, around the time when
primates started to split into two groups. Some of the retroviral
families found in humans arent found in New World Monkeys, such as
marmosets. Predecessors of the apes, including humans, were Old
World Monkeys, so Haussler and his team knew the retroviruses must
have made their attack after these primate lineages split. Ironically, the intrusion of these simple viral
packages of hereditary information could explain why we are so
different from our primate cousins. With their contribution to the
influence of molecules like p53, our fates are so entwined that we
as a species wouldnt be the advanced organisms we are today if it
werent for these ancient invaders. Our DNA is the very instruction
book that separates us from other species. Yet nearly one-tenth
of it comes from retroviruses, some of the simplest beings on the
planet. Ultimately, says Wang, that
shouldn't surprise us. Look at the human body. How much is human?
he says, his arms outstretched. There are 10 times more bacteria
[than human cells] if you just count the cells in our gut. Its
hard to say which part is foreign and which part is human. Top Sidebar: Virus vs.
Virus 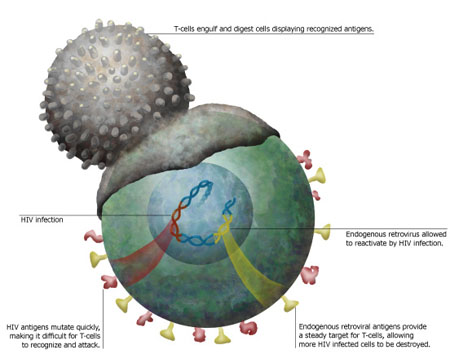 | Illustration: Kristy Whitehouse |
T cells constantly patrol the
body for dangerous invaders. These highly specialized guardians
of the immune system target specific pathogens. They even attack
endogenous retroviruses, the remnants of ancient infection that
survive within our genome, if the retroviruses spring into action.
Often, these usually dormant fossils alongside our genes will take
advantage of a suppressed immune system when an HIV infection takes
hold. A team of immunologists at UC San Francisco, now believes
that by exploiting the T cell response to this awakening, they may
be able to hone in on HIV itself. T cells
attack HIV just as they would any other virus. But one of the
problems they run into is that HIV changes, or mutates, so quickly.
By the time the body develops specific T cells to fight a particular
strain, the viruss minions have changed, making them unrecognizable
and thus impossible for the T cell to destroy. The immune system
is always playing catch up, says Keith Garrison, lead author of the
teams latest paper, published in PLoS Pathogens.
If the T cells had a steadier target, Garrison
and his colleagues hypothesized, they would form a more effective
battle line against HIV. T cells respond to proteins called antigens
on the surface of passing cells that indicate when a cell is infected.
A weakened, HIV-infected cell also is more likely to contain
reactivated endogenous retroviruses. While a T cell might not
recognize the antigen of shape-shifting HIV, an antigen prompted
by an endogenous retrovirus could prod other T cells into attack
mode. Healthy cells wouldnt show such activity, so T cells would
spare them. But T cells aimed at endogenous retroviruses would
destroy any cell with HIV. To test this
hypothesis, the group took blood samples from patients diagnosed
with HIV. They compared the number of T cells aimed at attacking
endogenous retroviruses with the amount of HIVwhat doctors viral
load. Individuals with more of that specific kind of T cell had
significantly less HIV present in their blood. The
findings suggest that rousing brigades of these targeted T cells
into action may slow the progression of HIV. To determine whether
T cell amplification could become a treatment option for the futureone
that could affect the design of a vaccinethe team is now searching
for the genetic factors that dictate whether an individuals cells
can control internal outbreaks of endogenous retroviruses.
A self-described retro-element biologist,
Garrison believes were only beginning to understand how big a role
endogenous retroviruses play. These fossils from within could
provide a weapon in the arsenal against HIV, but the research pathway
to get there will be long and challenging, he says.
Top Biographies John C. Cannon B.S.
(biology) The Ohio State University Internship: Los Alamos
National Laboratory news office Communication is essential in the natural world,
as I've seen throughout my research and global travels. Cells fired
cytokines back and forth to one another when I removed them from
their comfortable organ capsules in my immunology lab. Humpback
whales hung suspended in the Indian Ocean, catatonic save for
hour-long arias sung to woo potential mates hundreds of miles away,
as I listened from the deck of a scientific ship above them. A
mother cooed to soothe her colicky baby under a baobab tree on the
African Sahel, where I shared so many dinners during my Peace Corps
work. The science behind these stories is
captivating, and now its my turn to communicate. My challenge is
to transfer my excitement to readers, showing them thatas Kerouac
wrotethe circumstances of existence are pretty glorious. . . . . . . . . . . . . . . . . .
. . . . . . . . . . . . . . . . . . . . . .
. . . . . . . . . . . . Emily
Harrington B.S. (biology) Colorado
College After graduating with my
biology degree in 1998, I traveled and taught troubled youth in the
remote Utah wilderness. From this, I concluded that carrying heavy
packs, eating Top Ramen and sleeping on punctured Therma-rests
should only be done for pleasure, not for work. And so, I turned
my back briefly on science and ventured into art and graphic design.
I have now added a science illustration certificate to my suite of
skills and will be returning to my native Montana this summer to
sleep on Therma-rests, hike through the Rocky Mountains, and work
with the Biomimicry Institute in Missoula. .
. . . . . . . . . . . . . . . . . . . . . .
. . . . . . . . . . . . . . . . . . . . . .
. . . . . . Kristy Whitehouse B.S.
(biology, cellular/molecular concentration) Humboldt State University
Drawing has been a lifelong joy, but
always as a hobby. In school I was attracted to science, to learning
how things work and the processes that are used. Molecular biology
is especially interesting to me because proteins are mind-blowing
little organic machines. Visualizing how they behave instantly
takes me into a fascinating world of outer space mixed with deep
sea and a little physics. My constant drawing and proficiency in
science led others to bring the field of Science Illustration to
my attention, and I was hooked! Not only am I able to combine my
passions, I can also share this world with others and help them
understand what is going on in the often mysterious and complex
fields of genetics and proteomics. Top |